The New Spontaneous Generation?
“Early Complexity” Hypotheses for Primordial Life
Spontaneous generation was the long-held belief that some organisms could appear fully-formed from non-living matter. Maggots were believed to be generated by rotten meat. Mice were believed to be generated by rotten grain. No gods required. No millions of years of chemical and biological evolution. It could just happen. So the belief went.
The first major challenge to this idea came from Francesco Redi in 1668. Redi hypothesized that maggots grew not from rotten meat, but from eggs laid in the rotten meat by flies. He managed to demonstrate this by placing rotten meat in flasks, some covered with cloth and others not. Since the covered flasks did not permit flies to lay eggs in the meat, no maggots grew. Maggots grew only in the uncovered flasks.
Louis Pasteur delivered the final disproof of spontaneous generation in 1861 with the help of his handy swan-necked flasks. First he placed broth in a flask, boiled the broth, and let it sit. In a second experiment he did the same but with a neckless flask.
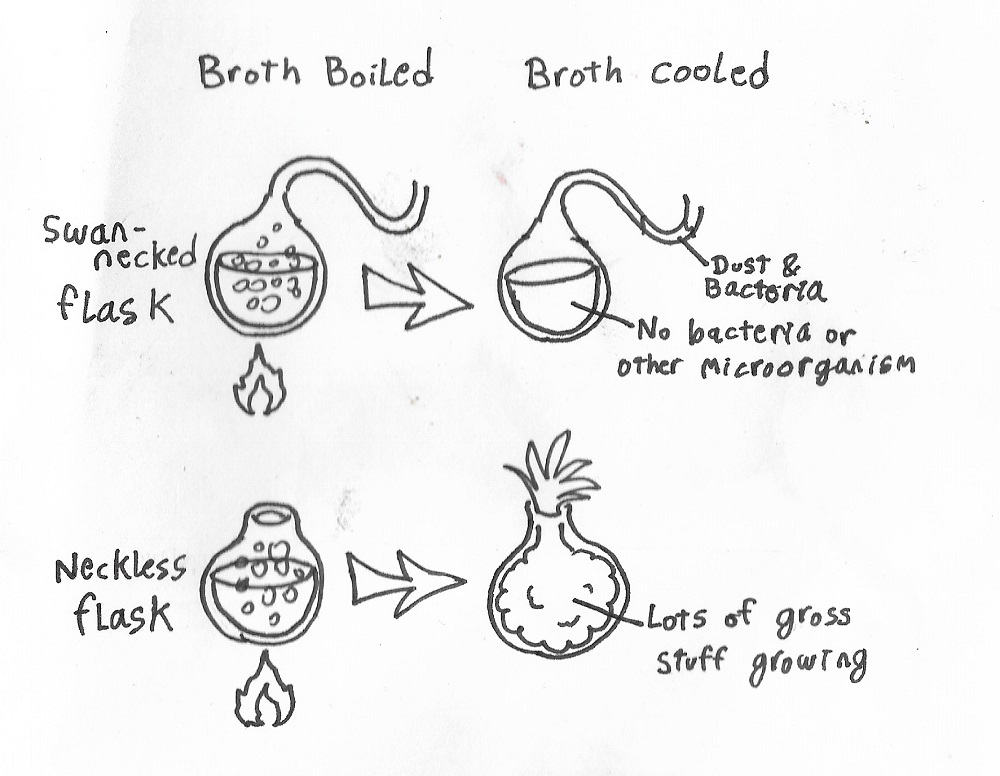
The swan-necked flask caught dust and microorganisms in the neck, but no microbes grew in the broth. The neckless flask, on the other hand, became the proud mother of a microbial ecosystem. This proved that air was not enough to get the broth to grow stuff in it. Spores and crap had to actually find their way into the liquid. Spontaneous generations was disproved.
Thus the principle of Biogenesis was established (Huxley, 1870): life, at least as it is known to us at present, can only be the result of previously-existing living things. The opposite doctrine, that life could arise from non-living matter, was termed Abiogenesis (Huxley, 1870). (Note that many have observed that the word biogenesis should properly mean “origin of life”, whereas abiogenesis should mean “non-origin of life”. These terms were derived somewhat abstractly. “Biogenic” means “from life”, so Huxley must’ve meant biogenesis to mean “origin from (within) life”, and then simply attached the a- suffix to mean the opposite, rendering abiogenesis as the term for “origin from non-life”.)
Although the idea that living things were being continually generated by the environment had been by Pasteur’s experiments thoroughly debunked, scientists were still left having to explain where life originated from to begin with.
In a letter to Joseph Dalton Hooker on February 1st, 1871, Charles Darwin said on the matter of abiogenesis that life may have originated in a "warm little pond”, containing “all sorts of ammonia and phosphoric salts, light, heat, electricity, &c., present, that a proteine compound was chemically formed ready to undergo still more complex changes."
Darwin also said in that same letter, "at the present day such matter would be instantly devoured or absorbed, which would not have been the case before living creatures were formed.”
In his book The Origin of Life, Alexander Oparin (1953) suggested that the "spontaneous generation of life" that had apparently been disproved by Louis Pasteur did occur at some point in the distant past, but that it was now impossible because the environmental conditions present on the early Earth had changed. Oparin also suggested in his 1953 book, much like Darwin before him, that any organism spontaneously generated today would immediately be predated by the organisms which pre-dated it.
The famous “prebiotic soup” experiment by Stanley Miller (Miller 1953, Miller-Urey experiment) had shown that by reacting a mixture of methane, hydrogen, ammonia and water in a spark discharge apparatus to simulate lightning, amino acids, the building blocks of proteins, could be formed along with other simple organic molecules (Moritz, 2010).
These conditions were chosen because observations of Jupiter and Saturn showed them to contain ammonia and methane, and hydrogen was inferred to exist in large quantities (Mortiz, 2010). These reducing atmospheres were thought to be remnants of the solar nebula and the atmosphere of the early Earth was assumed by analogy to have been similar (Mortiz, 2010). (Note that these experiments have since been shown to have simulated conditions more like that of the proto-planetary disk from which the Earth and the other planets formed (Hill & Nuth, 2003).)
Meteorites have shown higher-than-background levels of many key organic compounds, including amino acids, amphiphiles, and nucleobases, as well as inorganic phosphate. The purine bases adenine and guanine have been detected in meteorites as well, however the only pyrimidine-base compound formally reported in meteorites is uracil (Stoks & Schwartz, 1979), although cytosine cannot be ruled out (Shapiro, 1998; Peeters et al., 2003; Martins et al., 2006).
Ultraviolet irradiation of low-temperature H2O ices intended as astrophysical ice analogues has been shown to produce not only amino acids, quinones, and amphiphiles, but have also, with the introduction of pyrimidine, been shown to produce uracil (Nuevo et al., 2009), cytosine, and even thymine, however the abiotic synthesis of thymine is less straightforward compared to other pyrimidine-base compounds (Sandford et al., 2014). (That this would logically make the prebiotic synthesis of RNA easier than that of DNA could explain why RNA has a larger repertoire of functions than does DNA in modern cells.) Additionally, ribose and related sugars have been produced experimentally in astrophysical ice analogues (Meinert et al., 2016).
When RNA was discovered to have catalytic properties (Kruger &al., 1982), the RNA World hypothesis wasn’t far behind. This has only been reinforced by the now much-expanded catalytic repertoire of RNA, and its importance in key cellular reactions (Doudna & Cech, 2002) “which can be viewed as molecular fossils of an earlier world,” meaning we never fully transitioned out of this aforesaid “RNA World (Alberts et al., 2002).” Evidence indicating that the common ancestor of the Archaea and the Bacteria possessed an RNA-based genome (Leipe & al., 1999) and only after divergence each separately acquired DNA from DNA viruses, in which DNA first arose (Leipe & al., 1999; Forterre, 2002), has further reinforced this hypothesis.
Chemical evolution
There has been substantial work done showing the possible formation of natural “liposomes” (“bubbles” of fat that in many ways mimic cell membranes) in water.
Early Assumptions about the Last Universal Common Ancestor (LUCA) were, quite reasonably, that it must have had all of the features which today are common to all lifeforms. All modern organisms (except some viruses) have DNA, therefore the LUCA (of everything except viruses) probably had DNA. All modern organisms (except viruses) have cells, therefore, the LUCA (of everything except viruses) was probably a cellular lifeforms.
(Note: Some prefer to use the acronym LUCA to mean, “last universal cellular ancestor” to avoid having to say, “except viruses” all the time.)
When hydrothermal vent ecosystems were discovered, they were immediately glommed-onto as a potential model environment for the origin of life. (Note that this appears to be part of an overall pattern: bacteria are our most distant relatives and are assumed to be very similar to our most distant ancestors; hydrothermal vent ecosystems are as different as one can get from the environments modern humans inhabit and are assumed to be very similar to the environment of our most distant ancestors. Anything sufficiently “different” or “othery” automatically equals ancestral, for some reason.)
Doubt & Recent discoveries
Contrary to assumptions that the LUCA would’ve possessed DNA, the Last Universal Common Ancestor probably had an RNA-based genome (Leipe & al., 1999) and only after divergence each separately acquired DNA from DNA viruses, in which DNA first arose (Leipe & al., 1999; Forterre, 2002),
The Last Universal Common Ancestor may have been acellular (Martin & Russell, 2003; Koonin & Martin, 2005) (this idea was dealt a serious blow due to the identification of common membrane-encoding genes shared by all three domains (e.g., Forterre, 2014)).
Hydrothermal vents would’ve been far too hot for the earliest RNA segments to last very long. RNA hydrolizes very rapidly at room temperature or above (or indeed, at much above the freezing point of water) (Scoztak, 2012).
The optimal growth rate for the earliest natural “liposomes” is just slightly above the freezing point of water (Scoztak, 2012)
Dual phylogenic rRNA and protein analyses show that while the common ancestor of all modern bacteria was probably a thermophile (Boussau et al., 2008), meaning that it had an optimal growth temperature (OGT) around 65±15°C, and while the same was true for the common ancestor of all modern-day archaea (Boussau et al., 2008), the LUCA (the common ancestor of both groups) was probably a mesophile (preferred room temperature, OGT≤50°C) (Boussau et al., 2008) or even a cryophile (preferred cold, perhaps even near-freezing temperatures) (Scoztak, 2012). Meanwhile, hydrothermal vent hypotheses imply a hyperthermophilic progenote with an optimal growth temperature (OGT) ≥80°C.
Deep sea vents are therefore good potential environment for the earliest bacteria or for the earliest archaea, but not for the Last Universal Common Ancestor.
If we know so much about the gradual process of prebiotic synthesis leading origin of life, and from the first life to the Last Universal Common Ancestor, why then do so many recent off-beat and alternative origin-of-life hypotheses seem to be so reminiscent of spontaneous generation?
It has traditionally been assumed that the primordial lifeforms from which all modern life evolved would’ve been prokaryotic in nature. This assumption is probably due in part to anthropocentrism; those creatures which are most distantly related to us, we tend to see as the most primitive. There has been some push-back against the decidedly eukaryocentric notion that the bacteria and the archaea represent a more primitive stage of our own evolutionary history, in the form of “eukaryotes-first” hypotheses which postulate that the eukaryotic cell condition is the more ancestral.
In “eukaryotes-first” or “early eukaryotes” hypotheses for the origin of life, the Last Universal Common Ancestor is imagined to be at least a sort of “proto-eukaryoid” organism that had some form of nuclear-cytosol compartmentalization. Egel (2012) argues for a primitive form of nuclear-cytosol compartmentalization in the Last Universal Common Ancestor (LUCA), implying that this organism was therefore morphologically proto-eukaryoid.
Staley (2013) suggest that the Last Universal Common Ancestral State (LUCAS) was one of multiple nuclei sharing a single cytoplasmic matrix In complex “supercells” that in turn made up aggregate , colonial lifeforms (which might not have even fulfilled all of the criteria for being designated a lifeform!).
One hypothesis holds life on this planet began as a sort of planet-spanning “mega-organism” (Marshall, 2011). Carl Woese (1998) suggested that the Last Universal Common Ancestor (LUCA) of the three domains may antedate individuation into discrete lifeforms separated by cell membranes, and that the LUCA may have actually been a community of proto-cells with extremely primitive, porous membranes — called "progenotes" (Woese, 1998) — through which, due to pervasive lateral gene transfer (LGT) (Woese, 2002), genetic material was exchanged and propogated itself more-or-less freely in the form of virus-like replicons that used the progenotes as their habitat.
In all fairness, as weird as these “early eukaryotes” ideas are, they do have some things going for them. Bacteria have a higher reproductive rate than humans, which means they’ve gone through far more generations than we have since our common ancestor with them. Bacteria can therefore be described as far more evolved than we are. Yet, even though they are more evolved, they are far simpler morphologically than eukaryotes, especially obligately multicellular eukaryotes like ourselves.
Bacterial genomes show signs of extreme reduction. Many bacteria with smaller genomes are apparently descended from bacteria with larger genomes (Ochman, 2005). Bacterial genomes are highly condensed. Bacterial genomes are highly organized. Transcription and translation occur simultaneous in bacteria. Bacterial genomes have almost no introns (“junk DNA” that gets cut out of the RNA copy). Bacterial exons (the non-intron “keeper” bits of DNA) self-splice back together.
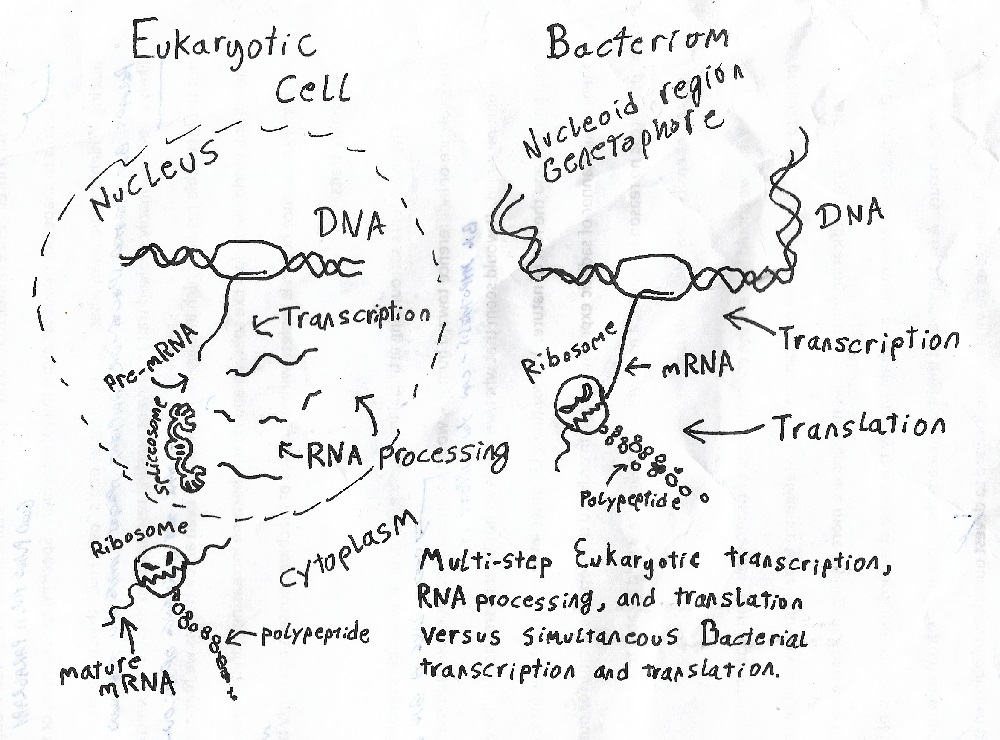
Bacteria have obviously been optimized by billions of years of natural selection to become the efficient, orderly organisms we know them as today (Ochman, 2005).
Eukaryotic cells are complex, bulky, inefficient, and overall far less advanced than their prokaryotic counterparts.
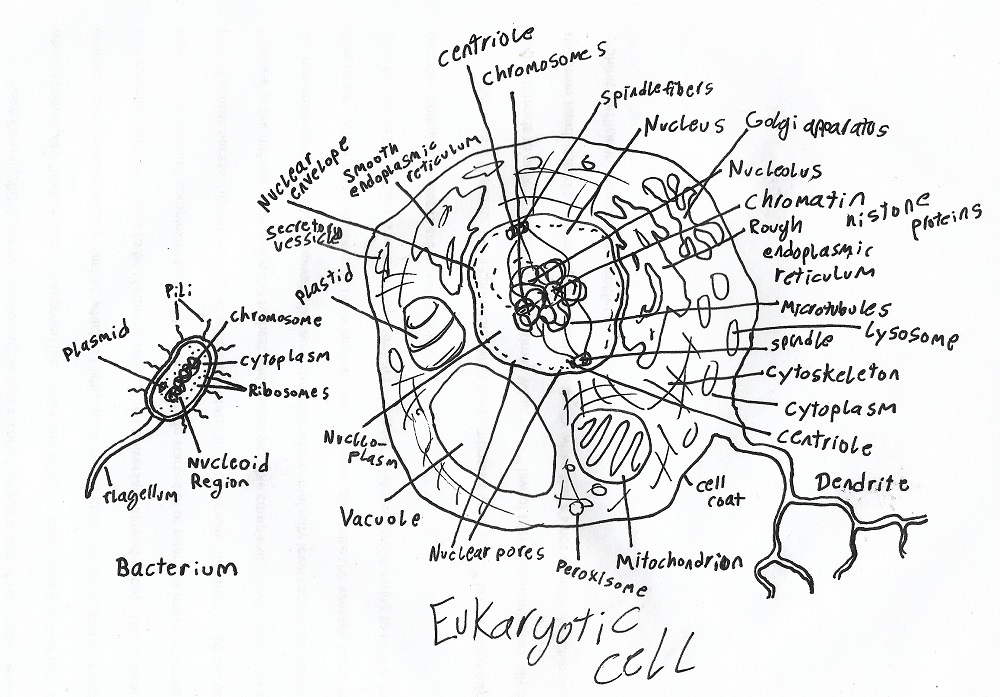
Furthermore, bacteria of the PVC superphylum (which includes the phyla Planctomycetes, Verrucomicrobia, and Chlamydiae as well as several others) have been demonstrated to have nuclear compartments, which could therefore be interpreted as an ancestral trait (Egel, 2013).
Another bit of evidence that lends credence to the early-complexity hypotheses is that the last universal common ancestor apparently had organelles (tiny cellular “organs” that do jobs for the cell). Not long ago, it was believed that only eukaryotes have organelles. In 2003, however, organelles called acidocalcisomes were discovered in bacteria (Marshall, 2011). Proteins for acidocalcisomes have been detected in the archaea and eucarya as well (Caetano-Anollés, 2011). This strongly implies that the last universal common ancestor had acidocalcisomes.
So it’s possible, from the evidence we’ve seen, that the last universal common ancestor had at least two organelles: a nucleus and a “proper” organelle (the nucleus is only counted as an organelle sometimes by some authors for some purposes, though it is often convenient to do so for the purposes of discussing things like serial-symbiogenic theory).
There are numerous problems with these early-complexity hypotheses, some more obvious than others.
The most obvious problem is the complete lack of support from the fossil record.
Hadean Eon — The earliest evidence of life thus far discovered on the planet are bacteria-like filaments from hydrothermal vent precipitates about 4.3 billion years old (Dodd, 2017). These were hydrothermal vent organisms. These were clearly prokaryotes (probably bacteria). (Remember earlier about the common ancestor of the archaea and the common ancestor of the bacteria both being hydrothermal vent organisms? This may be getting close to the LUCA!)
Archaean Eon (Palaeoarchaean & Mesoarchaean Eras) — The earliest direct evidence of cellular life comes from the archaea of this period (Schopf &al., 2018). The earliest evidence of any form of multicellularity from this time is from cyanobacteria about 3-3.5 billion years ago. Cyanobacterial stromatolites were formed by biofilms / microbial mats.
Proterozoic Eon (Palaeoproterozoic Era) — There is absolutely no evidence of eukaryotes prior to about 2.3 billion years ago. Some of the stromatolites from this period might have been made by early eukaryotic algaes.
There is no evidence of macroscopic complex obligately multicellular life prior to 2.1-2.2 billion years ago.
The fossil evidence conforms perfectly to the more orthodox “prokaryotes-first” model of primordial life. “Eukaryotes-first” and “mega-organism” models must excuse themselves with “unlikely to fossilize” or “incomplete fossil record”. They are speculations about what “might be” true, and depend on the relative lack of adequate evidence in the fossil record to say, “we don’t know for sure, so maybe this”. That’s fun to do, but it’s not good science.
Another obvious problem is that the eucarya seem to be a highly derived type of archaeon. After Woese introduced the 3-domain system in which all life was categorized as belonging to the archaea, the bacteria, or the eucarya, this was quickly challenged by the eocyte hypothesis (Lake &al., 1988), which posited that the eucarya are more closely related to some archaea than those archaea are to other archaea (in other words, we eukaryotes are nested within the archaeal family tree; we are not separate branches on the tree of life). This was followed by refinements/modifications to the eocyte hypothesis like the TACK hypothesis (Guy & Ettema, 2011) and more recently the “Asgardian” or Asgardarchaeota hypothesis (Eme &al., 2017), each nesting the eucarya more deeply within the archaea.
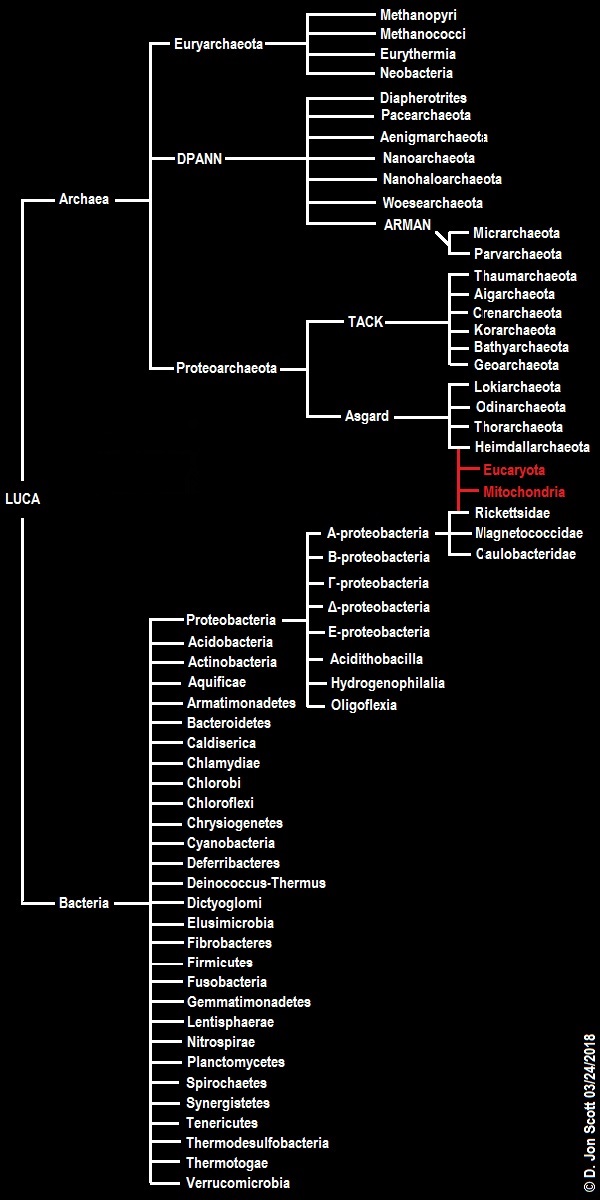
Given this datum, the only way to harmonize a “eukaryotes-first” hypothesis of origins with Asgard-nested eukaryotes is to propose that at least seven different lineages (see figure 4) must have “enucleated” (disposed of their nuclei) independently of one another. This is not necessarily a fatal flaw, as widespread parallel evolution when it comes to loss or reduction of features is not without evolutionary precedent (consider how during the Mesozoic era multiple lineages of theropod independently reduced the size of their forelimbs in order to make them more agile by reducing their overall rotational momentum), however it is one of the more obvious of the problems for a eukaryotes-first model to overcome and it does make it seem somewhat unlikely. A less obvious problem is the apparent dominance of prokaryotes over other forms of cellular life. In “Chance and necessity: the evolution of morphological complexity and diversity”, Sean B. Carroll (2001) advocates for a “passive” trend toward increasing biological complexity that implies the majority of living things today should be at roughly the same complexity level as the first organisms (or LUCA).
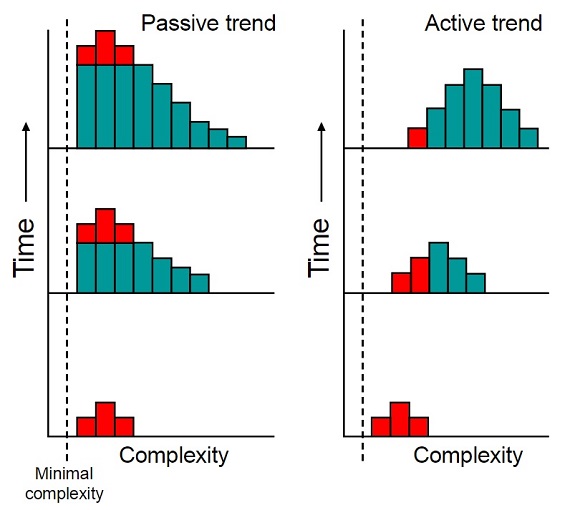
More complex forms would out-compete and supplant the simpler forms if there were an active trend toward increasing complexity (figure 3). The fact that prokaryotes greatly outnumber eukaryotes demonstrates that there has been no such active trend, which means that the majority of lifeforms on this planet should be more-or-less about as complex as the earliest lifeforms. This strongly suggests that the earliest lifeforms were prokaryotes, or if we consider viruses as lifeforms, something even simpler still. If the earliest lifeforms had been eukaryotes, we would expect creatures like slime-molds and algae to outnumber the prokaryotes – unless of course there has been an active trend toward greater simplicity, which isn’t entirely implausible on the face of it. (That is, after all, what natural selection will tend to do when it can; take the simplest, least-costly route.)
And of course no origin-of-life discussion would be complete without a mention of panspermia, or the idea that life on some planets (like Earth) could be or have been seeded by life from elsewhere (such as another planet, for example).
Molecular panspermia is the concept that some of the organic compounds necessary for life on Earth may have been delivered here from elsewhere. Lithopanspermia is the idea of fully-formed living things stowing away in rocks being flung through interplanetary (and even interstellar) space due to asteroidal and cometary impacts. Interplanetary lithopanspermia refers to cells or organic molecules being transported by rocks across interplanetary space, or between planets within a single planetary system.
In the case of Mars-Earth interplanetary lithopanspermia, either key organic compounds like RNA may have formed first on Mars and been transported to Earth, or else actual, cellular lifeforms made the trip.
Many microbial organisms have been shown to be able to survive a trip through space. We know for example that bacteria could have survived being ejected into space by planetary impacts (Stoffler et al. 2006), survive the subsequent rapid acceleration (Mastrapaa et al. 2001), survive exposure to vacuum and high radiation (Saffary et al., 2002; Bucker & Horneck, 1970; Horneck, 1971; Nicholson, et al. 2005), thrive and grow in asteroidal and meteoritic interiors (Mautner 2002), survive re-entry into a planetary atmosphere (blah) and subsequent impact (blah).
Interstellar lithopanspermia — The statistical probability of panspermia is difficult to ignore. Considering how much space dust falls to Earth every year, the frequency with which Earth is hit by asteroids, the Sun’s course through the Milky Way, &c., &c., and the fact that even very small grains of dust might contain whole colonies of microbial organisms, it is highly probably that we’ve spread Earth microbes all over the galaxy (Wallis, 2003; Napier, 2003). This also makes it very unlikely that the Earth was the ultimate benefactor of this process rather than one of the practically infinite beneficiaries (Napier, 2003).
This is perhaps the most damning thing of all for early-complexity hypotheses with regard to whether the first lifeforms on Earth were complex, yet may also be the only way that the LUCA could realistically have been complex. The 4.2 billion-year-old organisms we’ve discovered look very much like what we would expect from either the common ancestor of the archaea or the common ancestor of the bacteria, but not what we would expect from the common ancestor of both. Since the Earth is only 4.55 billion years old, that doesn’t give much time for the complex common ancestor in the eukaryotes-first hypotheses to have evolved from something simpler. So the possibility that it didn’t evolve here but was transported from elsewhere could provide a way around this problem. Unfortunately, it creates another huge problem in that it’s very unlikely that a morphologically complex organism would’ve been capable of surviving a trip through interplanetary space. However, if the Earth is 4.55 billion years old and biological life can be traced back at least 4.2 billion, and it looks like the modern domains had already formed by then, then it’s entirely possible that the archaea/bacteria split had already occurred before the Earth had formed. This would mean that the archaea and the bacteria could’ve arrived here separately from one or more source locations.
Conclusion
Both the traditional “prokaryotes-first” model of common descent and adherents to various religious mythologies have proclaimed that biological complexity must be exceedingly difficult, therefore requiring either millions of years of evolution (for the traditional scientific view), or the divine hand of a supernatural entity (for mythology), these “early complexity” hypotheses seem to stand apart in implying that complexity might be easier than previously thought. In this way, the early complexity hypotheses can be seen as having something in common with spontaneous generation, as both are “complexity is easy” scenarios which stand in contrast to the more orthodox “complexity is hard” doctrines of creationism and traditional common descent scenarios.
If these ideas turned out to be true, it wouldn’t require as great an attenuation to our concept of how evolution precedes as one might think. We’ve grown accustomed to thinking that the first lifeforms must’ve been at or near the “complexity bottom floor” for life on Earth, yet this completely ignores the fact that absent a significant amount of excess complexity, these first lifeforms would also be nigh-perfectly efficient. With eukaryotes or even super-organisms appearing before prokaryotes, we would instead be faced with a situation where the last common universal ancestor was big, clumsy, inefficient, cluttered with all kinds of excess complexity and unable to reproduce itself in full. Efficiency, on the other hand, rather than complexity, would be the feature which required evolutionary “work”. In both scenarios, primordial lifeforms are missing a key component that makes modern organisms successful: In “early efficiency” scenarios, the primordial prokaryotes are missing the complexity that allows a small minority of organisms to flourish; in “early complexity” scenarios, the early eukaryotes are missing the efficiency that allows the majority of organisms to flourish. This is merely an argument over which feature is the more difficult to evolve: complexity or efficiency. And as with all things in science, the answer will be borne out by the evidence, not by any of our preconceptions about what “must” be true.
References
Ayala, FJ. (2007) Darwin’s greatest discovery: design without a designer. PNAS 104(1) https://www.ncbi.nlm.nih.gov/books/NBK254313/
Bastien Boussau, Samuel Blanquart, Anamaria Necsulea, Nicolas Lartillot & Manolo Gouy. Parallel adaptations to high temperatures in the Archaean eon. Nature 456, 942-945 (18 December 2008) doi:10.1038/nature07393 https://www.nature.com/articles/nature07393
Life Sci Space Res. 1970;8:33-8. Survival of microorganisms under simulated space conditions. Bucker H, Horneck G. https://www.ncbi.nlm.nih.gov/pubmed/11826891
Carroll, SB. (2001) Chance and necessity: the evolution of morphological complexity and diversity. Nature 409. https://www.nature.com/articles/35059227
Dodd, MS, Papineau, D, Grenne, T et al. (5 more authors) (2017) Evidence for early life in Earth’s oldest hydrothermal vent precipitates. Nature, 543 (7643). pp. 60-64. ISSN 0028-0836 https://doi.org/10.1038/nature21377
Doudna JA, Cech TR. The chemical repertoire of natural ribozymes. Nature. 2002 Jul 11;418(6894):222-8. DOI:10.1038/418222a
Forterre, P. “The Common Ancestor of Archaea and Eukarya Was Not an Archaeon” Archaea. Volume 2013 (2013), Article ID 372396, 18 pages http://dx.doi.org/10.1155/2013/372396 Received 22 July 2013; Accepted 24 September 2013
Life Sci Space Res. 1971;9:119-24. Survival of bacterial spores under some simulated lunar surface conditions. Horneck G, Bucker H, Wollenhaupt H. https://www.ncbi.nlm.nih.gov/pubmed/12206178
Le Page, M. (2008) Evolution myths: Natural selection leads to ever greater complexity. New Scientist. https://www.newscientist.com/article/dn13617-evolution-myths-natural-selection-leads-to-ever-greater-complexity/
Detlef D. Leipe L. Aravind Eugene V. Koonin. Did DNA replication evolve twice independently? Nucleic Acids Research, Volume 27, Issue 17, 1 September 1999, Pages 3389-3401, Published: 01 September 1999 https://doi.org/10.1093/nar/27.17.3389
Mariscal, C. & Doolittle, WF. (2015) Eukaryotes first: how could that be? Philos Trans R Soc Lond B Biol Sci. 360(1678) https://www.ncbi.nlm.nih.gov/pmc/articles/PMC4571562/
Marshall, M. (2011) Life began with a planetary mega-organism. New Scientist. https://www.newscientist.com/article/mg21228404-300-life-began-with-a-planetary-mega-organism/
Mautner, Michael N. Planetary Resources and Astroecology. Planetary Microcosm Models of Asteroid and Meteorite Interiors: Electrolyte Solutions and Microbial Growth- Implications for Space Populations and Panspermia. Astrobiology. Volume 2, Number 1, 2002. Mary Ann Liebert, Inc. Research Paper
Mastrapaa, R.M.E.; Glanzberg, H.; Head, J.N.; Melosh, H.J.; Nicholsonb, W.L. Survival of bacteria exposed to extreme acceleration: implications for panspermia. Earth and Planetary Science Letters 189 (2001) 1^8. Received 12 December 2000; received in revised form 9 March 2001; accepted 10 April 2001
Napier, W. M. A mechanism for interstellar panspermia. Mon. Not. R. Astron. Soc. 348, 52-61 (2004). Accepted 2003 October 2. Recieved 2003 October 1; in original form 2003 April 4. doi:10.1111/j.1365-2966.2004.07287.x http://onlinelibrary.wiley.com/doi/10.1111/j.1365-2966.2004.07287.x/abstract
Ochman, H. (2005) Genomes on the shrink. PNAS-USA 102(34) https://www.ncbi.nlm.nih.gov/pmc/articles/PMC1189353/
Roya Saffary, Renu Nandakumar, Dennis Spencer, Frank T Robb, Joseph M Davila, Marvin Swartz, Leon Ofman, Roger J Thomas, Jocelyne DiRuggiero. Microbial survival of space vacuum and extreme ultraviolet irradiation: strain isolation and analysis during a rocket flight. FEMS Microbiol Lett. 2002 Sep 24;215(1):163-8.10.1111/j.1574-6968.2002.tb11386.x http://onlinelibrary.wiley.com/doi/10.1111/j.1574-6968.2002.tb11386.x/full
J. William Schop Kouki Kitajima,d Michael J. Spicuzza,d Anatoliy B. Kudryavtsev,b and John W. Valleyd SIMS analyses of the oldest known assemblage of microfossils document their taxon-correlated carbon isotope compositions PNAS 2018 Jan 2; 115(1): 53–58. Published online 2017 Dec 18. doi: 10.1073/pnas.1718063115
Shapiro, R. Prebiotic cytosine synthesis: A critical analysis and implications for the origin of life. (1999) PNAS, vol. 96 no. 8, 4396-4401. (received for review November 19, 1998)
Dieter Stoffler, Gerda Horneck, Sieglinde Ott, Ulrich Hornemann, Charles S. Cockell, Ralf Moeller, Cornelia Meyer, Jean-Pierre de Vera, Jorg Fritz, Natalia A. Artemieva. Experimental evidence for the potential impact ejection of viable microorganisms from Mars and Mars-like planets. Icarus 186 (2007) 585-588 Received 12 July 2006; revised 3 November 2006; available online 21 December 2006
Available online @ http://www.sciencedirect.com/science/article/pii/S0019103506004143
Stoks P. and Schwartz A. Uracil in carbonaceous meteorites. Nature, 282, 709 (1979)
https://www.nature.com/articles/282709a0
Jack Szostak: Reconstructing the First Cells. MoleCluesTV. Youtube. 2011
https://www.youtube.com/watch?v=rAZTiWZU6Cc
Jack Szostak (Harvard/HHMI) Part 1: The Origin of Cellular Life on Earth. iBiology. Youtube. Published on Jan 11, 2012
https://www.youtube.com/watch?v=PqPGOhXoprU
Jack Szostak (Harvard/HHMI) Part 2: Protocell Membranes. iBiology. Youtube. Published on Jan 11, 2012
https://www.youtube.com/watch?v=CJ5jh33OiOA
Jack Szostak (Harvard/HHMI) Part 3: Non-enzymatic Copying of Nucleic Acid Templates. iBiology. Youtube. Published on Jan 11, 2012
https://www.youtube.com/watch?v=jfq5-i8xoIU
Jack Szostak: Origin of life on Earth and design of alternatives. MoleCluesTV. Youtube. 2017
https://www.youtube.com/watch?v=rAZTiWZU6Cc