The 2nd Law of Thermodynamics
What it Really Means for Evolution
The second law of the thermodynamics states that when energy is used, unusable energy must increase, and that the amount of unusable energy or “entropy” in a system can never decrease. Physical and chemical interactions tend to break complex molecules down into simpler ones as once-usable energy is dispersed throughout the system and is for all intents and purposes lost. This is often paraphrased as systems tending to move from orderly to disorderly, or as things going from complex, ordered states to simple, disordered states.
A common creationist claim is that since the evolution of life on Earth requires that today’s highly ordered, complex lifeforms developed from humbler, simpler origins, evolution therefore contradicts one of the fundamental laws of reality. The stock response to this is to state that the 2nd law of thermodynamics only applies to closed systems, while neither the Earth nor biological life are closed to their surroundings (both receive large amounts of energy from the Sun, for example), and that local decreases in disorder are allowed as long as there’s still an increase in disorder for the larger system. Another common response is to point out that the common paraphrase of the 2nd law is misleading to laypersons; the words “order” and “disorder” don’t mean the same thing in the context of thermodynamics that they mean to everyday people, leading some to criticize usage of the terms (e.g., Lambert, 2002)
While creationists have come up with various counter-responses to these arguments, and we’ve developed counter-counterarguments, I’d like to attack this from a slightly different angle: The evolutionary history of life on Earth depends on the 2nd law of thermodynamics such that the 2nd law could even be said to in a sense “predict” increases in biological complexity over time.
Going back to the embryonic years of evolutionary theory, Lamarck (1809, in McShea, 1991) believed that lineages tend to increase in complexity over time (he believed this was due to “invisible fluids” that lay dormant in the environment and find their way into living organisms, modifying tissues and opening up canals, eventually forming whole new organs). The effect of the environment, on the other hand, according to Lamarck, was mainly to retard this process of “complexification”. There were thus two “dueling forces” in evolution: An “adaptive” force, which serves to streamline and simplify organisms over time, and a “complexifying” force, which tends to make organisms more complex.
Because the history of life on Earth was viewed rather anthropocentrically at the time, scientists of the 19th century tended to view evolution as a process which slowly transformed simple, bacteria-like lifeforms into large, complicated organisms like ourselves. When Charles Darwin managed to convincingly demonstrate natural selection was the primary mechanism for evolutionary change, the natural conclusion was that the overall trend in evolution toward more complex forms must therefore be because complex organisms are inherently more fit. Darwin himself expressed reservation about this as it seemed to him that simpler, more efficient forms should have a decided advantage over larger, more complex, more reproductively lethargic forms (McShea, 1991). Nevertheless, natural selection was the only realistic explanation for evolutionary change known at the time and creatures had clearly become more complex.
The idea of an internal drive toward more complex forms, termed “orthogenesis” (Haacke, 1893), eventually became a taboo subject in the scientific community when Ernst Mayr politically assassinated the term in the journal Nature in 1948 by stating that it implied "some supernatural force" (Ruse, 1996).
Later, in the mid-to-late 1980s, it was suggested that increases in biological complexity might be an essentially thermodynamic process having to do with accumulation of entropy (Salthe, 1985 and Wicken, 1987 in McShea, 1991). Natural selection, on the other hand, often simplifies organisms over time, and complexity might only arise when selective pressure is low or absent (Ayala, 2007 & Le Page, 2008).
This is not difficult to imagine. Bacteria reproduce far more quickly than we do, and have thus experienced far more generations since our common ancestor with them. They are therefore far more evolved than we are, and they are in many ways elegantly, breathtakingly simple and efficient: genes which code for similar functions are adjacent to one another in bacterial genomes (meaning quicker access to genes that will next be used, and related genes are more likely to travel together when bacteria swap genes); genetic information is transcribed from DNA to messenger RNA and messenger RNA is translated into proteins simultaneously rather than going through the comparatively the slow, multi-step process seen in eukaryotic cells; they rarely bother with introns (“junk DNA” that gets cut-out of the RNA copy before molecular machines called “ribosomes” translate the RNA into proteins) compared to eukaryotes and when they do, their RNA self-splices back together again rather than requiring the spliceosomes (“splice-o-somes”) employed by eukaryotic cells.
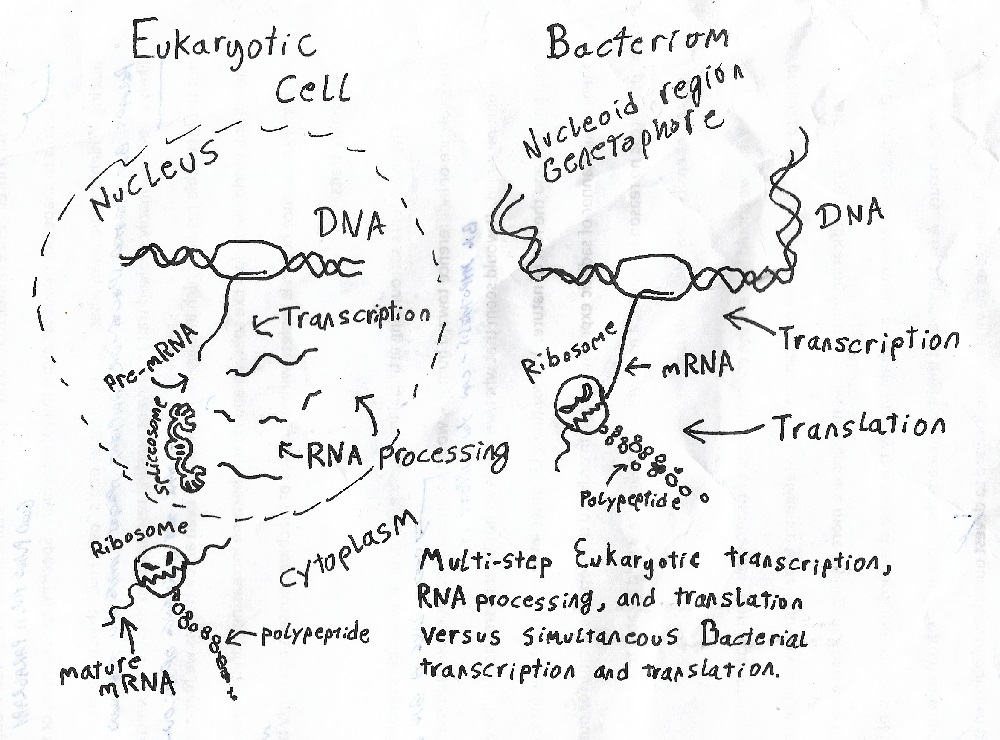
Figure 1 – Complex eukaryotic transcription & translation (left) versus streamlined bacterial transcription & translation (right). While bacterial transcription & translation can’t be truly described as simpler in any absolute sense (for they have more functions going on simultaneously at any given time and are in that way doubly complex as compared to eukaryotes), the process is definitely much-streamlined and has completely done away with complex steps. (Image by D. Jon Scott, © 2019)
These guys have clearly been honed by billions of years of natural selection to become the simplest, most orderly, most efficient they could be (Ochman, 2005). Eukaryotic cells clearly have not. (I would even go so far as to say the existence of eukaryotic cells falsifies the “intelligent design” hypothesis – unless of course the designer was David Cronenberg!)
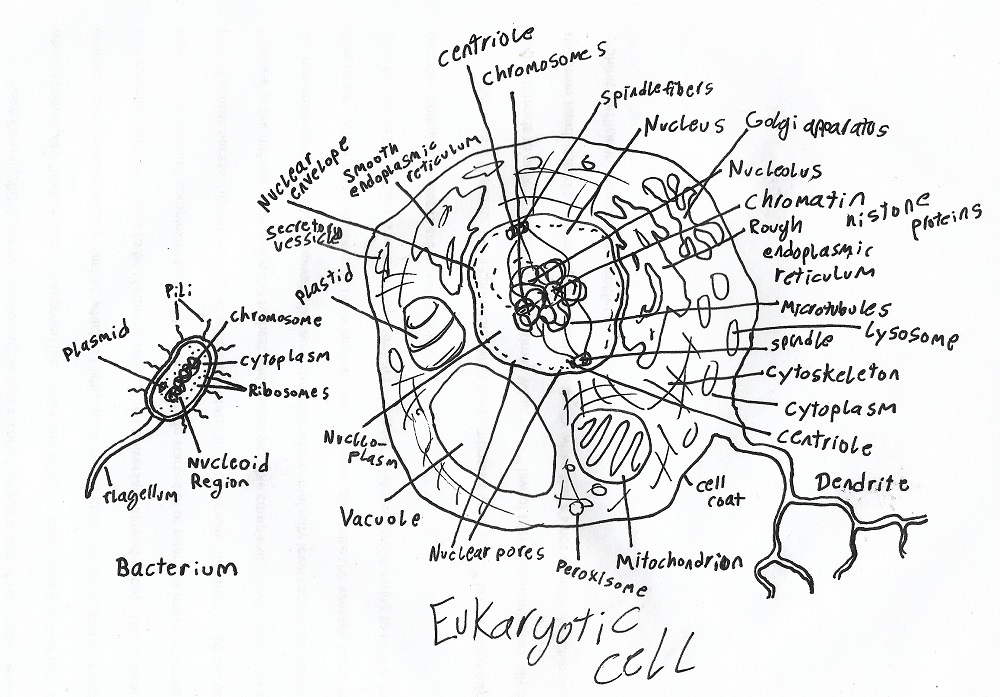
Figure 2 – Sensible, well-ordered bacterial cell (left) versus highly offensive Eukaryotic “Cronenberg” cell (right). (Image by D. Jon Scott, © 2019)
It’s even possible the Last Universal Common Ancestor (LUCA) of all of life on Earth was in many ways more similar to modern plants and animals than to modern bacteria (Egel, 2012; Staley, 2013; Mariscal & Doolittle, 2015). Egel (2012) suggests that the common ancestor of the eukaryotes and the bacteria had a primitive form of nuclear-cytosol compartmentalization and was therefore anatomically proto-eukaryoid in its morphology. Staley (2013) argues that the LUCAS was one of “super cells” – multiple nuclei sharing a single cytoplasmic matrix – and that these supercells in turn made up large, colonial, aggregate lifeforms (which may not yet have even been “true” lifeforms in the strictest sense of the word!). If such a “super-eukaryoid” LUCAS did exist, one may speculate that the only reason eukaryotic cells continue to get away with their excessive complexity / inefficiency on a planet ruled by prokaryotes is that the proto-eukaryote managed to domesticate a bacterial symbiont into the modern mitochondrial battery that powers our cells today.
One hypothesis even holds that “Life began with a planetary mega-organism” (Marshall, 2011).
Still, even if we assume the traditional view that the LUCA was far more like a bacterium than like a eukaryote (let alone some kind superorganism), it would be a stretch to say that the overall average complexity level for life on Earth has increased.
Sean B. Carroll (2001) argues for a “passive” trend toward increasing biological complexity as a sort of statistical inevitability when a complexity “bottom floor” is imposed because there is “nowhere to go but up”: Any increase in the difference between the highest and lowest possible complexity levels could only push the maximum complexity level upward, because the minimum complexity level cannot be pushed downward. While it has occasionally been implied that this would also raise the average complexity level for life on Earth, Carroll portrays the number of “simple” lifeforms proliferating to such a degree that the “complex” lineages are a statistically insignificant and possibly ever-diminishing percentage of the total number of living things.
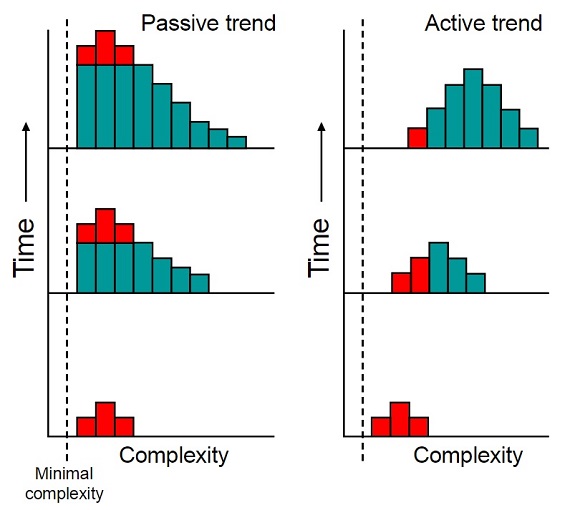
Figure 2 – A passive trend toward complexity (left) versus an active trend toward complexity (right). With a passive trend, the starting complexity level of living things continues to be the complexity level for the majority of living things, while the most complex lifeforms are always the least numerous. (Image by Tim Vickers, Public Domain)
With an active trend toward complexity, the more complex forms out-compete and begin to supplant the simpler forms (figure 3). Being that that bacteria and archaea both vastly outnumber eukaryotes, and that single-celled eukaryotes vastly outnumber multi-celled eukaryotes, any trend toward complexity has clearly been a passive one; a “statistical side-effect” of the proliferation of prokaryotes.
One problem with this “passive trend” hypothesis is that it strongly suggests that the primordial lifeforms that everything evolved from should be roughly as simple as the majority of living things today, whereas that does not appear to be the case. Bacteria with smaller genomes consistently appear to be descended from bacteria with larger genomes (Ochman, 2005). This means that the majority of organisms alive today are simpler than the bacteria of yesteryear (which harkens back to the “eukaryotes-early” and “supercell” hypotheses discussed earlier). Carroll (2001) observes that the genetic diversity in the natural world comes nowhere near reflecting the number of genetic combinations possible by genetic drift alone, and suggests that natural selection has imposed significant limits on genetic diversity (duh!). That natural selection is the primary limiting force on diversity and has clearly, strongly selected for simplicity such that most organisms today, the archaea and the bacteria, are simpler than ancient bacteria, strongly implies that natural selection is doing exactly what the creationists claim the 2nd law of thermodynamics should do.
This is not to say that the 2nd law never works as a limiting factor, however. Common sense tells us that as systems break down, efficient systems will tend to outlast inefficient ones. This means that there should be an overall tendency to move from a large number of relatively inefficient systems to a smaller number of more efficient systems (this could be regarded as a sort of natural selection). What the creationists want the 2nd law to do is to universally break things down and make them less efficient over time, which is roughly the effect that living things tend to have on their environments, and why complex organisms can prosper when there is an environment suitable to support them. Conversely, this may also explain why larger, more complex organisms (those which have accumulated the most entropy) are the first to die off in times of environmental stress.
This case is made even stronger when we consider viruses as lifeforms. Viruses are unable to reproduce on their own and parasitize true, cellular lifeforms in order to reproduce. It is fair to assume they’ve been simplified well below the “complexity floor” for cellular life and are likely far simpler than the first lifeforms would have been even if life began at the simplest possible state it could exist in (without parasitizing other lifeforms, that is). Since viruses are the dominant biological entities on this planet, considering them as living things would mean that no matter what the first lifeforms were, prokaryote or “super-eukaryote”, these first living things were undoubtedly more complex and less efficient than most living things today.
So it appears that natural selection tends to make things simpler and more efficient over time, while the 2nd law of thermodynamics leads to increasing biological complexity when there’s not enough selective pressure to prohibit complex, inefficient systems from proliferating.
References
Ayala, FJ. (2007) Darwin’s greatest discovery: design without a designer. PNAS 104(1) https://www.ncbi.nlm.nih.gov/books/NBK254313/
Egel, (2013)
Carroll, SB. (2001) Chance and necessity: the evolution of morphological complexity and diversity. Nature 409. https://www.nature.com/articles/35059227
Lambert, FL. (2002) Disorder – a cracked crutch for supporting entropy discussions. J. Chem. Educ. 79(2) https://pubs.acs.org/doi/abs/10.1021/ed079p187
Le Page, M. (2008) Evolution myths: Natural selection leads to ever greater complexity. New Scientist. https://www.newscientist.com/article/dn13617-evolution-myths-natural-selection-leads-to-ever-greater-complexity/
Mariscal, C. & Doolittle, WF. (2015) Eukaryotes first: how could that be? Philos Trans R Soc Lond B Biol Sci. 360(1678) https://www.ncbi.nlm.nih.gov/pmc/articles/PMC4571562/
Marshall, M. (2011) Life began with a planetary mega-organism. New Scientist. https://www.newscientist.com/article/mg21228404-300-life-began-with-a-planetary-mega-organism/
McShea, DW. (1991) Complexity and evolution: what everybody knows. Biology & Philosophy. 6(3) https://link.springer.com/article/10.1007/BF00132234
Ochman, H. (2005) Genomes on the shrink. PNAS-USA 102(34) https://www.ncbi.nlm.nih.gov/pmc/articles/PMC1189353/
Staley, (2013)